This webpage describes our team’s efforts to develop metabolic glycoengineering (MGE) technology relevant to tissue engineering and exploit it for regenerative medicine applications. First, in Section 3.1, we describe the development of MGE monosaccharides analogs that install thiol groups into the outer periphery of the glycocalyx, where these versatile chemical functional groups are ideally positioned to interact to their microenvironment including both natural and artificially engineered growth substrates. In Section 3.2 we describe proof-of-concept experiments where this approach was used to create adhesive interfaces for Jurkat cells, which are lymphoma cells that normally grow in suspension. Next, in Section 3.3, we extend this technology to show how MGE promotes neuronal differentiation through Wnt signaling in human embryonic cells and, in Section 3.4, describe how this approach can be extended to human neural stem cells (hNSCs) useful for repairing spinal cord and brain injuries. Finally, in Section 3.5 we describe pioneering experiments extending thiol-based MGE to human adipose stem cells (hASCs).
3.1 Introduction: Designing and Validating a Glycoengineered Cell:Material Interface
3.1.1 Metabolic Glycoengineering Thiol Groups into Cell Surface Glycans
We designed Ac5ManNTGc, a ManNAc analog designed to installed thiol-modified sialic acids in the glycocalyx (Figure 3.1.1):
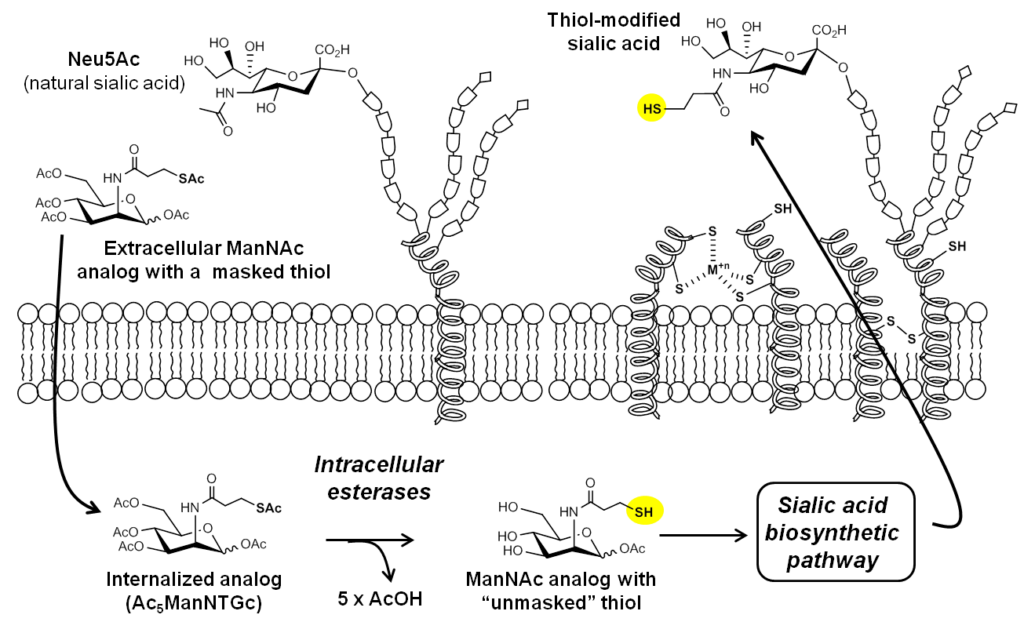
The design and synthesis of Ac5ManNTGc is described by Sampathkumar et al, 2006; flow cytometry methods to quantify the expression of cell surface thiols are also described in Nature Protocols.
3.1.2 Visualization of Cell Surface-displayed Thiols
As mentioned above, cell surface expression of thiol functional groups can be quantified by flow cytometry. The increased thiol display on Ac5ManNTGc-treated cells can also be visualized by staining the cells with thiol-reactive fluorophores or, as shown in Figure 3.1.2, by quantum dots designed to be thiol-reactive:
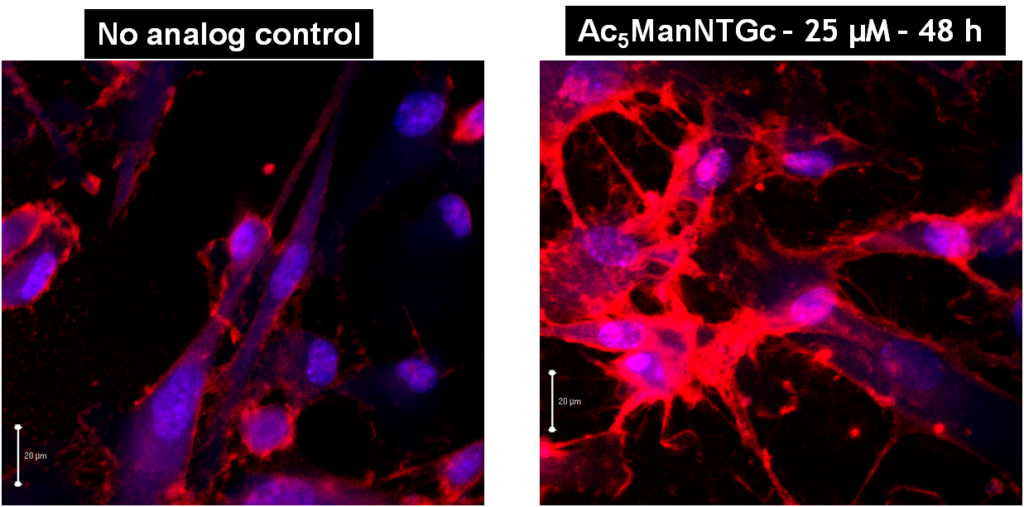
As shown (Figure 3.1.2, left), untreated cells have a low background level of reagent-accessible thiol groups, which is expected due to the presence of cysteines in cell surface proteins. Upon treatment with Ac5ManNTGc, staining for thiol groups increase about 10 to 15-fold (Figure 3.1.2, right). Upon reduction of the cells with DTT, the signal increases by another order of magnitude (i.e., to ~100 to 130-fold higher than untreated control cells), indicating that most Ac5ManNTGc-installed cell surface thiols exist in the oxidized, crosslinked forms and therefore are inaccessible to labeling reagents.
3.2 Proof-of-Concept Experiments in Cancer Cells
3.2.1 Attachment of MGE’d, Glycothiol-Displaying Jurkat Cells to Complementary 2D Surfaces
Upon demonstrating that Ac5ManNTGc increases the surface display of thiol groups able to interact with a cell’s microenvironment (in Section 3.1, above), we sought to exploit these thiol groups to “build in” new forms of cell adhesion to control cell fate. As a first step in this direction, we used Jurkat cells because this cancer cell line is normally non-adhesive and we reasoned that creating an adhesive binding interface would serve as a proof-of-principle experiment to demonstrate our proposed thiol-based binding interface. Upon treating these cells with Ac5ManNTGc we achieved selective adhesion to thiol-reactive chemical functional groups including pyridyldithiols, maleimide, and gold (a maleimide-derivatized surface is shown in Figure 3.2.1).
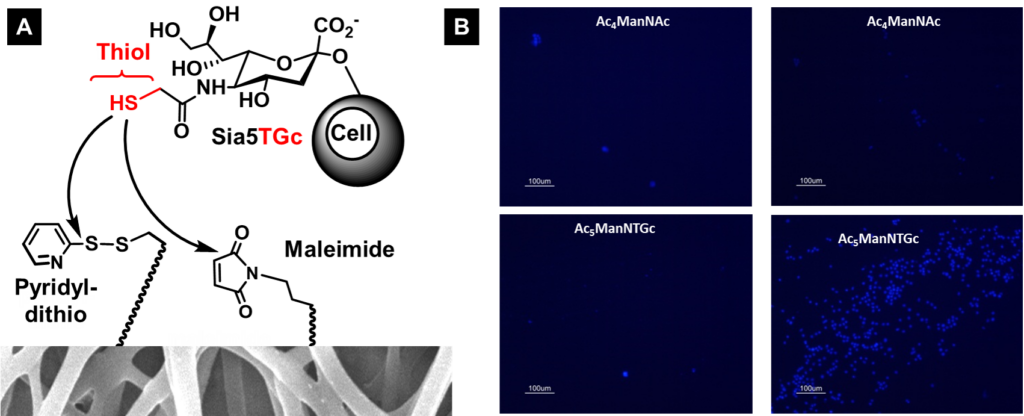
3.2.2 Attachment of MGE’d, Glcyothiol-Displaying Jurkat Cells to 3D Scaffolds
Upon demonstration that a binding interface could be designed that enabled the normally non-adhesive Jurkat cell line to attach to a chemically complementary, maleimide-derivatized 2D substrate after treatment with Ac5ManNTGc (Figure 3.2.1, above with results shown for a gold surface shown below in Figure 3.2.2[A}), we tested gain-of-adhesion in three dimensions. In these experiments, we coated PES nanofibers with gold and tested the adhesion of untreated control (Figure 3.2.2[B], top and Ac5ManNTGc-treated (Figure 3.2.2[B], bottom) Jurkat cells.
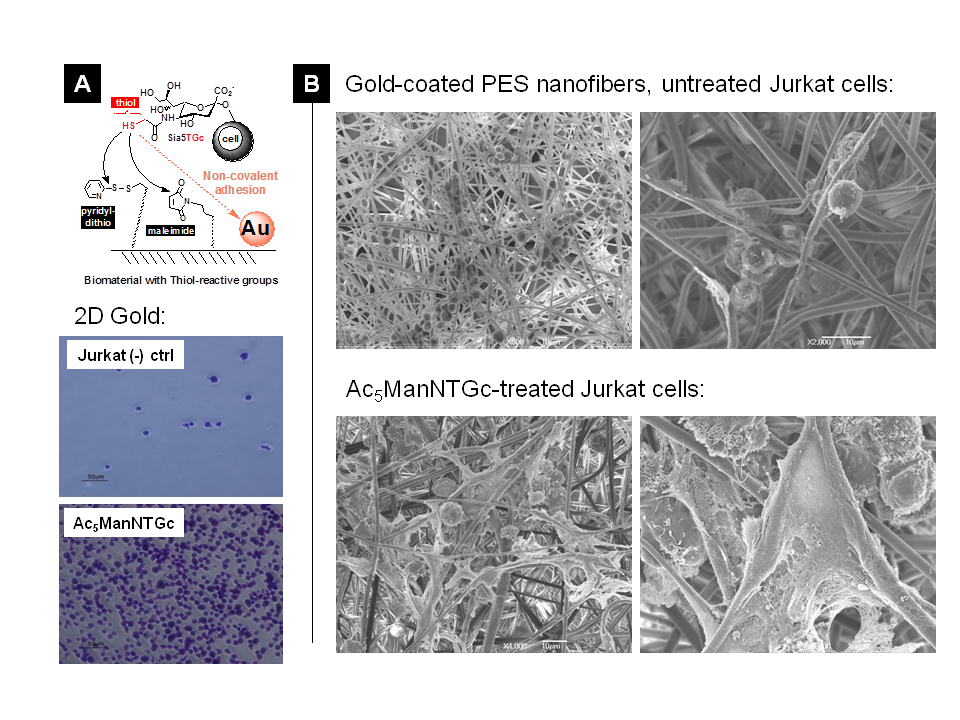
The 3D adhesion experiment was noteworthy for two reasons; one was that gain-of-adhesion for the normally non-adhesive Jurkat line was achieved in the absence of Ac5ManNTGc treatment but instead depended on the topology of the substrate. Second, there nevertheless was a unique gain-of-function endpoint observed in the thiol-expressing Jurkat cells, which was the copious production of extracellular matrix components. Additional information on this experiment is published (by Du et al., 2011).
3.3 Glyco-control of Neuronal Differentiation in Human Embryonic and Stem Cells
3.3.1 Background
One goal of applying thiol-based metabolic glycoengineering (Figure 3.1.1, above) was to “build in” cell adhesion (e.g., as shown in Figure 3.2.1 for Jurkat cells) to facilitate that attachment of cells to biomaterials for tissue engineering applications. The observation that a metabolically glycoengineered (MGE’d) binding interface also could control cell fate (Figure 3.2.2) provided a complementary facet for using thiol-based analogs in regenerative medicine. Specifically, the exploit the ability shown in cancer cells suggested that we could control cell fate in stem cells by modulating their differentiation outcomes (Figure 3.3.1).
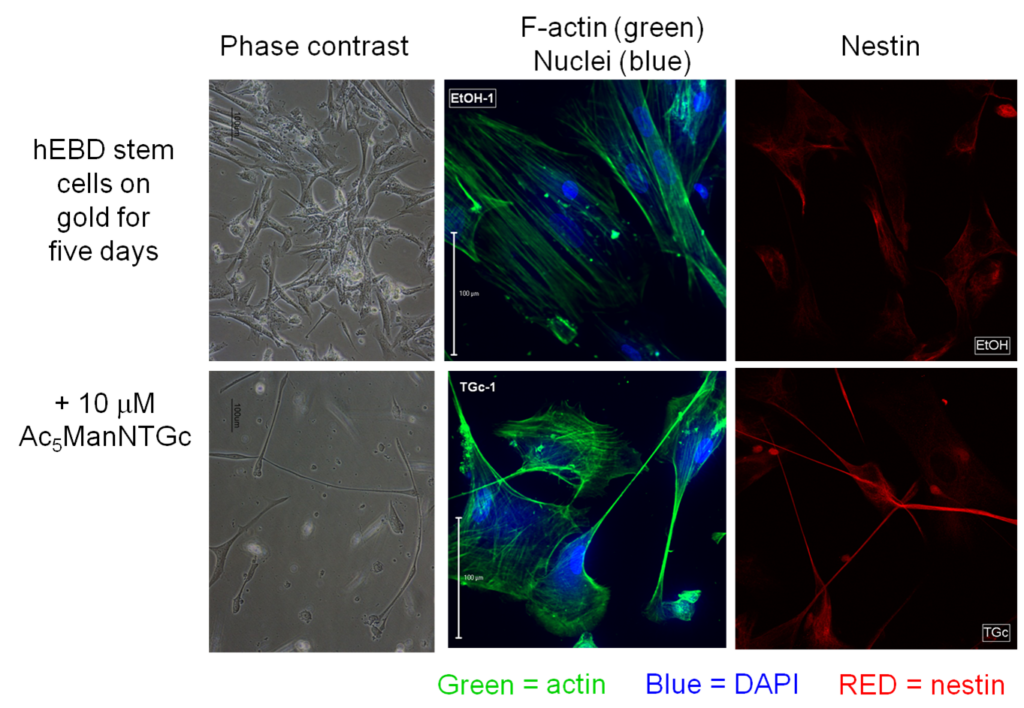
3.3.2 Neuronal Differentiation in Glycothiol-MGE’d Human Embryotic Cells involves Non-Canonical Wnt Signaling
To confirm neuronal differentiation occurred as suggested by the responses shown above in Figure 3.3.1, we demonstrated the accumulation of beta-catenin in Ac5ManNTGc-treated cells, which is a biochemical marker / requirement of differentiation of cells towards neural cell types (Figure 3.3.1).
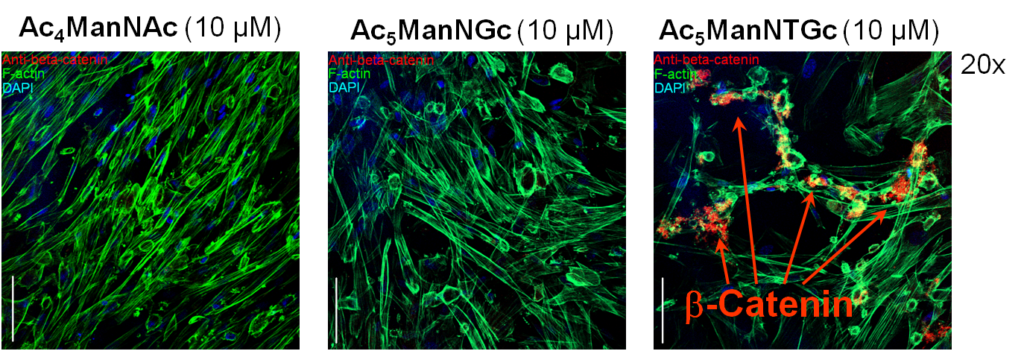
In this experiment, all cells were grown on a 2D gold substrate, resulting in beta-catenin accumulation when the cells were treated with Ac5ManNTGc; similar accumulation did not occur when the cells were grown on collagen (the normal growth substrate for the hEBD cells used in this experiment) or for cells treated with Ac4ManNAc (left panel) or Ac5ManNGc (the non-thiol “glycolyl” version of Ac5ManNTGc).
3.4 Enhanced Cell Adhesion and Differentiation in Human Neural Stem Cells (hNSCs)
Coming soon
3.5 Impact of Glycoengineering in Human Adipose Stem Cells (hNSCs)
Coming soon